By: Nijas Kunju, Technical Manager in Application Engineering, ANSYS Inc
India’s robust investment in the aviation sector, exemplified by the substantial budgetary allocation of $72 billion for defense aviation in 2023, with $20 billion of domestic earmarking, points to an era of growth and innovation for the sector. This strategic investment has catalyzed the emergence of a vibrant private sector engagement in the development of cutting-edge drones, UAVs, and HAPS (high-altitude platform systems), complementing the government’s initiatives on 5th and 6th-generation fighter aircraft.
This transformative landscape includes initiatives such as the Indian Defense Offset and Make in India programs, fostering an environment conducive to heightened global participation in India’s Aerospace and Defense sector. Leading Aerospace and Defense OEMs, recognizing the imperative of technological advancement to maintain competitiveness, are steadfastly embracing modern innovations to elevate their product offerings.
With burgeoning market demands and a compelling investment climate, stakeholders increasingly advocate for expedited time-to-market for advanced stealth vehicles. Achieving this goal necessitates a paradigm shift towards minimizing prototype iterations and striving for “first-time-right” design success. Herein lies the pivotal role of Digital Engineering solutions, seamlessly integrating data management, Model-based System Engineering, and multidisciplinary analysis and optimization through physics-based simulations. Embracing these cutting-edge methodologies expedites product development and ensures enhanced agility and precision, propelling the Aerospace and Defense industry into unparalleled innovation and efficiency.
Prevailing challenges
Developing defense technologies involves strict requirements and limitations. In military aircraft, achieving stealth capability is crucial to evade detection by radar and other detection methods. This involves shaping the aircraft to minimize its visibility on radar and reducing its visual, acoustic, and infrared signatures. However, creating a stealthy design has drawbacks, including compromises in aerodynamic performance, longer development times, reduced fuel capacity, higher maintenance needs, and higher costs.
Among the various stealth technologies, radar avoidance is critical because radar can detect aircraft from long distances, regardless of weather or time of day. Therefore, reducing the aircraft’s radar cross-section (RCS) is a primary focus. Several methods exist, such as smoothing sharp edges, changing the aircraft’s shape (which can affect aerodynamics), using radar-absorbing materials (which can pose challenges at high speeds and specific frequencies), and employing active RCS cancellation techniques.
Advanced electromagnetic simulations, such as Finite Element Method (FEM), Finite Difference Time Domain (FDTD), Integral Equation (IE), physical optics (PO), and Signature-based Reduction (SBR), are used to virtually implement and accurately predict the RCS of aircraft structures (Figure 1-2).
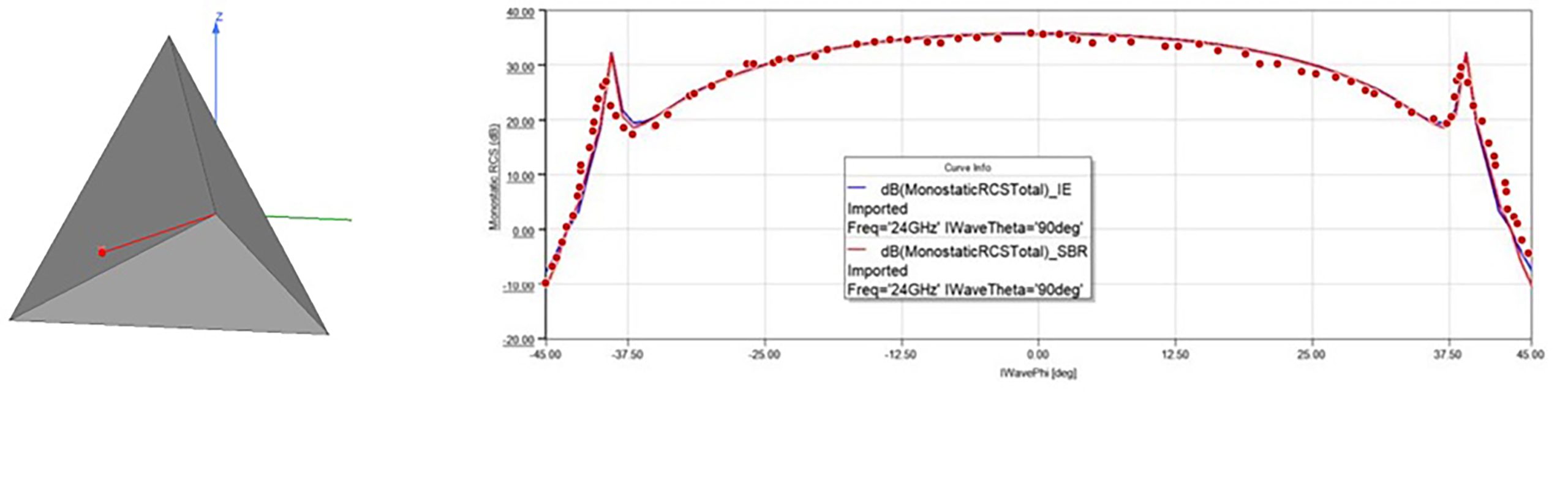
Navigating the complexities of identifying areas on aircraft or vehicles that contribute to high RCS poses a significant challenge for designers. Implementing effective mitigation strategies, such as employing radar-absorbing paints and structures, realizes pinpoint accuracy in identifying these problematic regions. Leveraging advanced 2D and 3D ISAR imaging techniques provides invaluable insights into these critical areas. Figure 3 vividly illustrates that the RCS spikes dramatically when electromagnetic energy aligns with an incident wave direction of approximately +/-98 degrees. The ISAR image comprehensively depicts these high-return regions, empowering designers to select and apply optimal RCS reduction methods strategically.
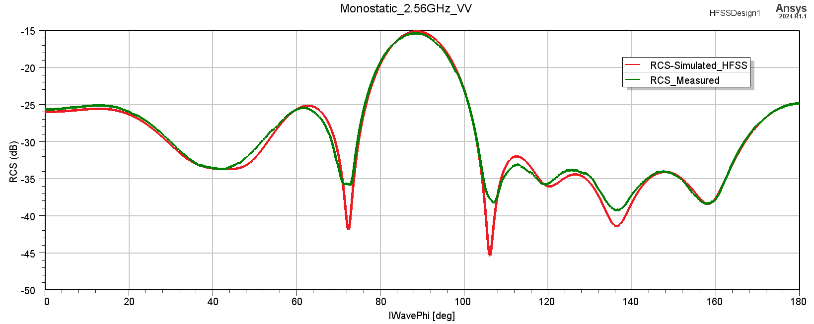
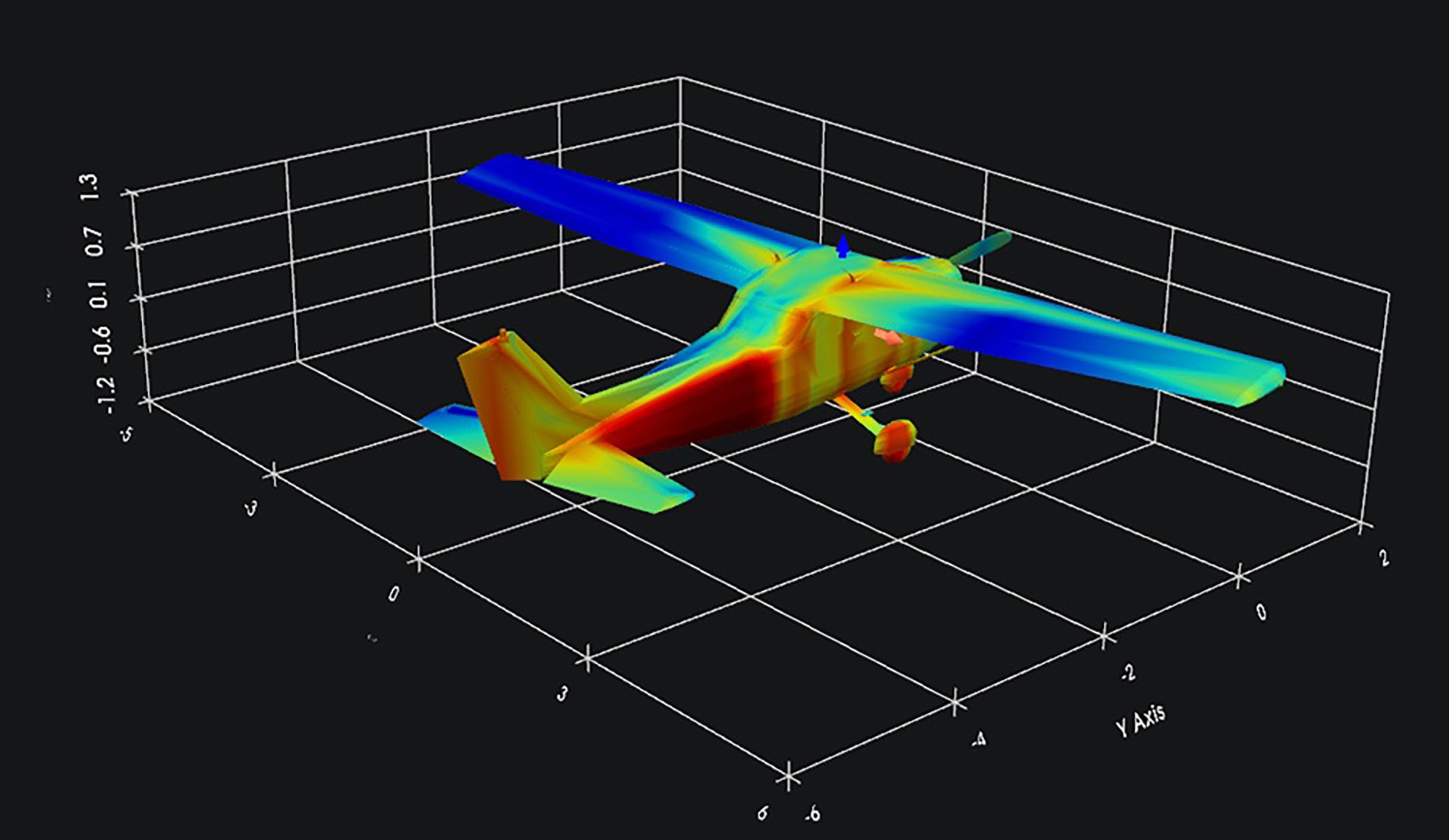
Transforming the defense landscape through stealth technology
Our airborne devices are protected by stealth technology, but the need for advanced radar systems to identify and classify targets remains just as important. Different types of radar are used to identify targets: bistatic, monostatic, etc. More recently, there has been an increase in the use of low-flying unmanned aerial vehicles (UAVs) and drones for defense applications. Distinguishing them from birds or other civilian objects requires high-resolution radar with a sophisticated signal processing system that can extract specific features of the target movement. AI/ML methods for classification are also being used, such as ISAR imaging, micro-Dopple effects, etc. However, these AI/ML methods require a large set of training data for each target type, such as drones, birds, UAVs, etc. For example, you need training data from different radar perspectives while in flight to identify a drone. One also needs different radars with different operating frequencies and bandwidths to capture the full spectrum of the target signatures. However, this diversity in radar systems makes it challenging to acquire comprehensive training data.
Advanced simulations can produce synthetic data mirroring real-world object behavior through numerical computation techniques. This facilitates the virtual recreation of electromagnetic properties at target frequencies and radar antennas, resulting in simulated radar raw IQ data. Users can use this raw data directly or apply their signal-processing algorithms to the simulated data. The processed data then serves as input for training AI/ML models [Figure 4]. The accompanying image depicts the detection of a drone using a 40GHz radar with a range resolution of 0.1 meters (achieved through a bandwidth of 1499 MHz). The Range-Doppler image illustrates the Doppler shift produced by rotating the drone’s front and back blades. Since rotating blades have components moving towards and away from the radar, they generate positive and negative Doppler velocity spreads in the spectrum.
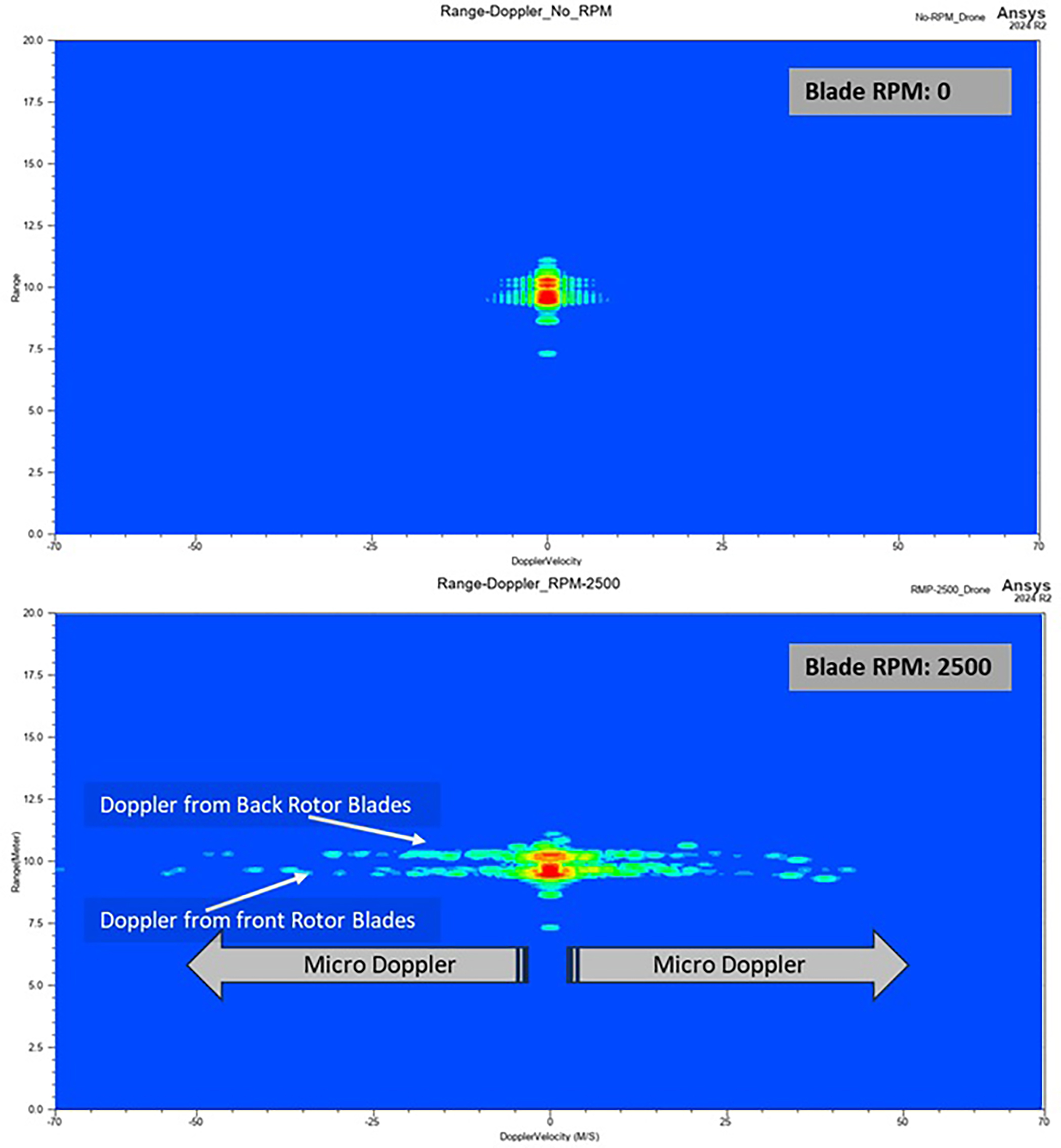
Observing a target over an extended duration (approximately 100-200ms) and analyzing its micro-Doppler signature can extract additional details such as rotor blade speed. This process assumes the target has already been identified using AI/ML techniques applied to the Range-Doppler image [Figure 5].
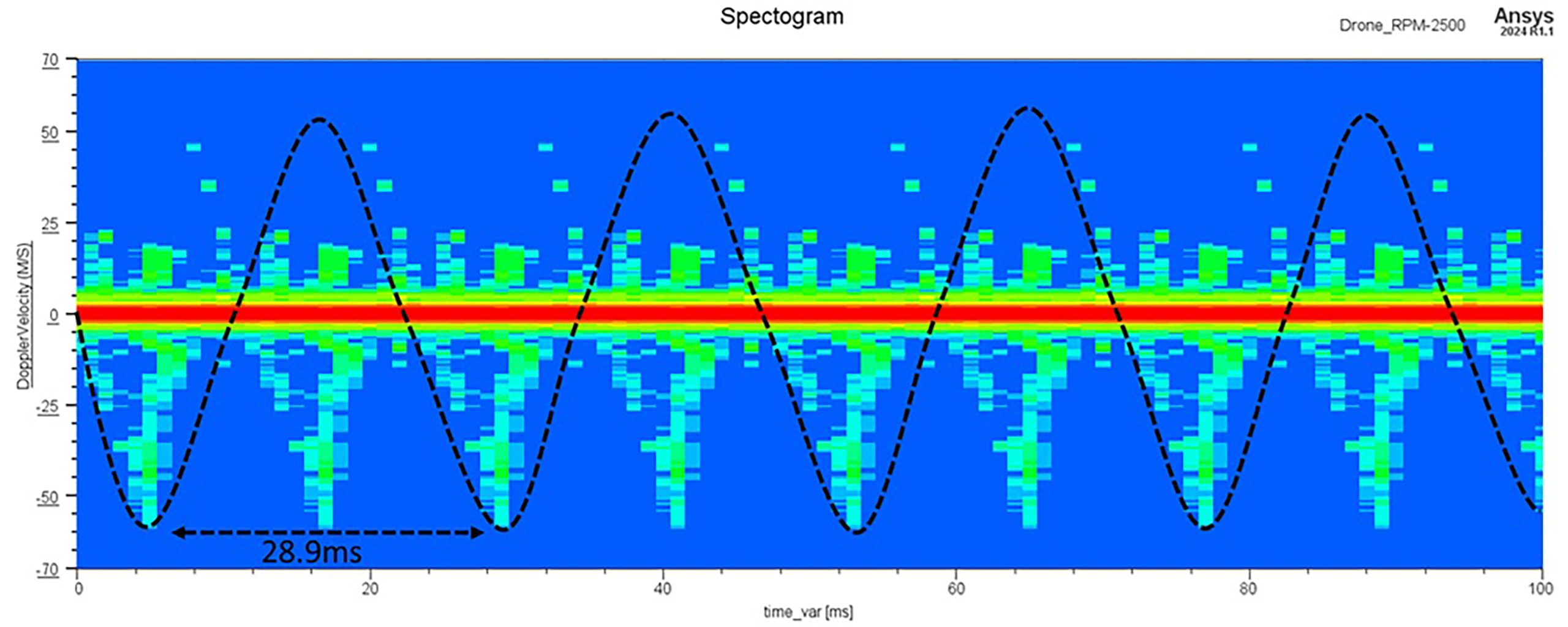
To summarize, many challenges will continue to permeate, pushing the boundaries of innovation in this ever-evolving landscape of advanced stealth and radar technology. Yet, within these challenges lie unparalleled opportunities to redefine the capabilities of modern defense systems and the future of warfare. As we strive to overcome obstacles such as detection evasion and signal manipulation, we are compelled to harness the full potential of emerging technologies and interdisciplinary collaboration. To fulfill the market demand today, digital missions and high-fidelity behavioral models, virtual twins are the way of the future. Organizations such as Ansys are collaborating closely to expedite the development of products, aiming to bring this vision to reality. We will only unlock new frontiers in stealth and radar technology through perseverance, ingenuity, and technological innovations, paving the way for enhanced security and strategic advantage for India in an increasingly dynamic world.